
You can inflate the floor up to 15 psi for the best performance with heavier loads in rough water. Made of tough drop-stitch PVC material, the Freestone inflates to a very rigid 9 psi, creating a stout 14-foot drift boat.The 6" diameter ports have a removable screw-on cover that lets you operate the boat as a non-bailer. There are two drain ports in the drop-stitch floor one in the center and one near the stern.The 14' Freestone's smaller size and lower hull height make it highly maneuverable in technical water and capable in windy conditions.The self-bailing inflatable hull handles rocks and shallow water like a raft while tracking as stealthily as a traditional drift boat.It's perfect for those technical streams where the fishing's great but a rigid drift boat hull isn't practical. Hess F (1978) Lifting-surface theory applied to ship-rudder systems.The 14' NRS Freestone Drifter is inflatable and includes a break-down frame system. J Soc Naval Architects Jpn 110:31–42 (in Japanese) J Marine Sci Technol 24(1):1–15įujii H, Tuda T (1961) Experimental research on rudder performance (2).
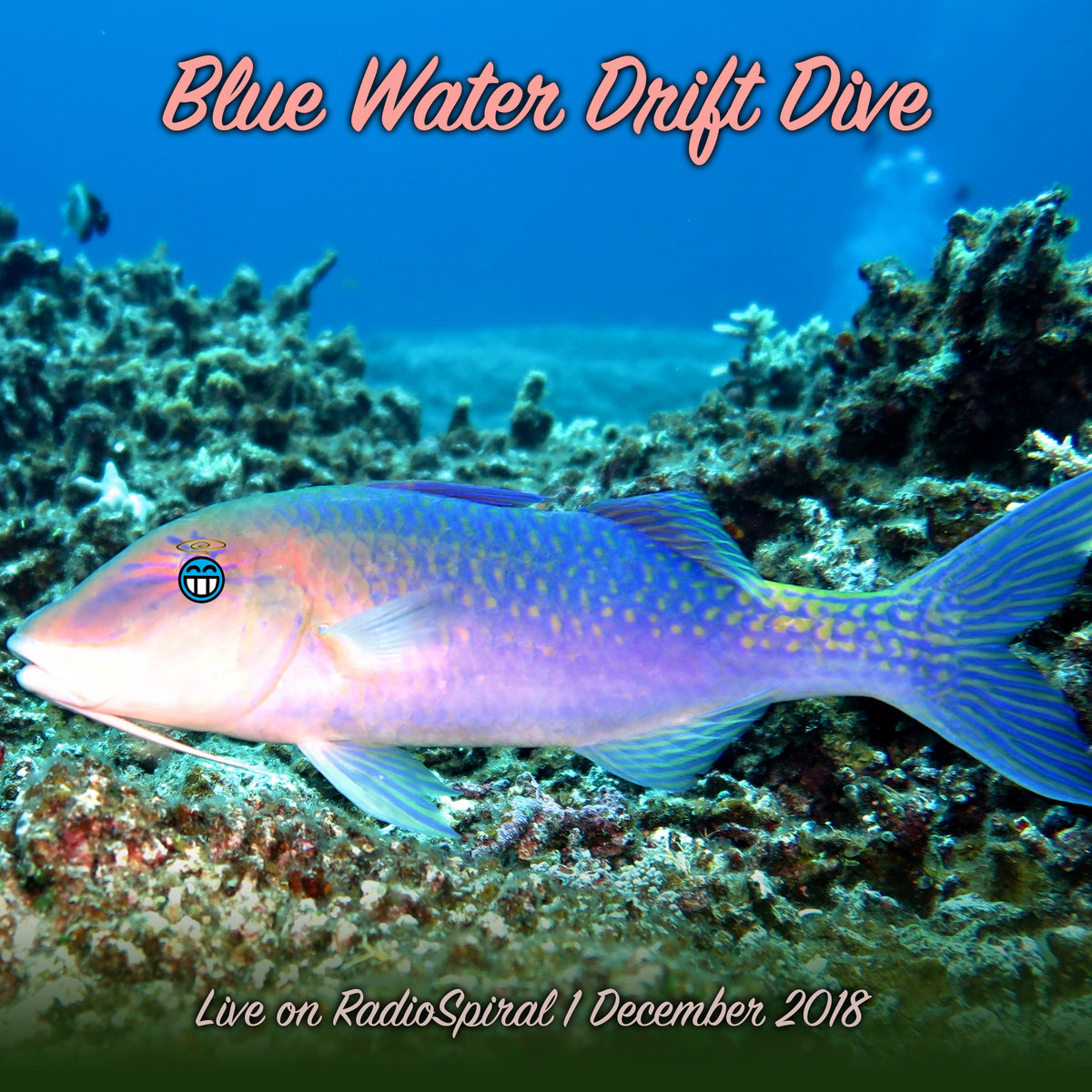
#Ship water drift full
Yasukawa H, Hirata N, Matsumoto A, Kuroiwa R, Mizokami S (2019) Evaluations of wave-induced steady forces and turning motion of a full hull ship in waves. 4th International Conference on Ship Manoeuvring in Shallow and Confined Water with Special Focus on Ship Bottom Interaction (4th MASHCON), Hamburg, pp 273–286 J Kansai Soc Naval Architects 209:91–101 (in Japanese)ĭelefortrie G, Eloot K, Lataire E, Van Hoydonck W, Vantorre M (2016) Captive model tests based 6 DOF shallow water manoeuvring model. Yumuro A (1988) Some experiments on maneuvering hydrodynamic forces in low speed condition. Sutulo S, Guedes Soares C (2015) Development of a core mathematical model for arbitrary manoeuvres of a shuttle tanker. international conference on ship manoeuvrability –prediction and achievement, Vol.1, paper 10 Kobayashi E, Asai S (1987) A simulation study on ship manoeuvrability at low speed. J Soc Naval Architects Jpn 160:93–102 (in Japanese) Takashina J (1986) Ship maneuvering motion due to tugboats and its mathematical model.

15th Symposium on Naval Hydrodynamics, Hamburg, pp 83–108 Oltmann P, Sharma SD (1984) Simulation of combined engine and rudder manoeuvres using an improved model of hull-propeller-rudder interactions. J Soc Naval Architects Jpn 155:132–138 (in Japanese)
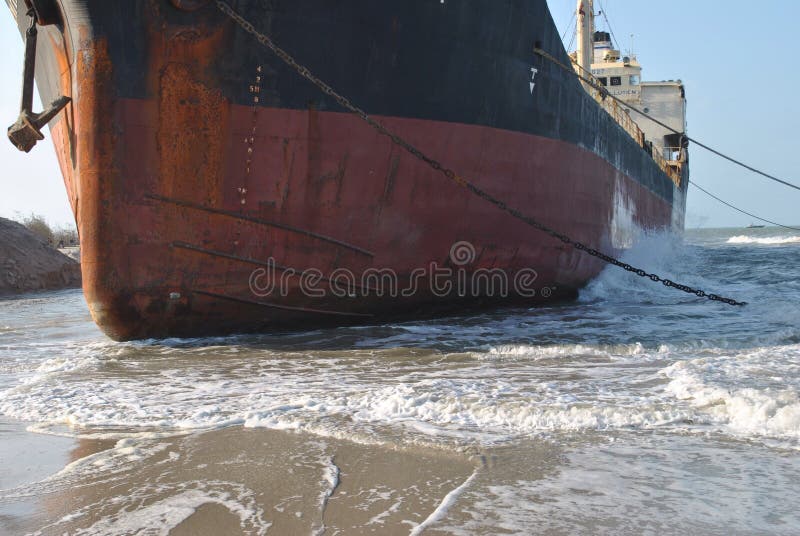
Kose K, Hinata H, Hashizume Y, Futagawa E (1984) On a mathematical model of maneuvering motions of ships in low speed. Yasukawa H, Yoshimura Y (2015) Introduction of MMG standard method for ship maneuvering predictions. Ratio of wake coefficients at propeller and rudder positions in new formula Ratio of wake fraction at propeller and rudder positions ( \(=(1-w_R)/(1-w_P)\)) \(\varepsilon _n\) Rudder aspect ratio ( \(=H_R^2/A_R\)) \(\kappa \)Īn experimental constant for expressing \(u_R\) \(\kappa _n\)Īn experimental constant for expressing \(u_R\) in new formula \(\nabla \)ĭisplacement volume of ship \(\rho \) Ratio of propeller diameter to rudder span ( \(=D_P/H_R\)) \(\varLambda \) Rudder angle where rudder normal force becomes zero \(\eta \) In the tests, hydrodynamic forces acting on the ship, the rudder normal force, and propeller thrust are measured while changing the hull drift angle \(\beta \) in the range of \(-180^\)įlow straightening coefficient \(\delta \) The rudder force, including interference with a ship hull in large drifting conditions, is investigated in this study by captive model tests using a ship model with steering.
